SpaceX
There are many challenges involved in a mission to Mars. What are they and what technology is SpaceX working on to address them?
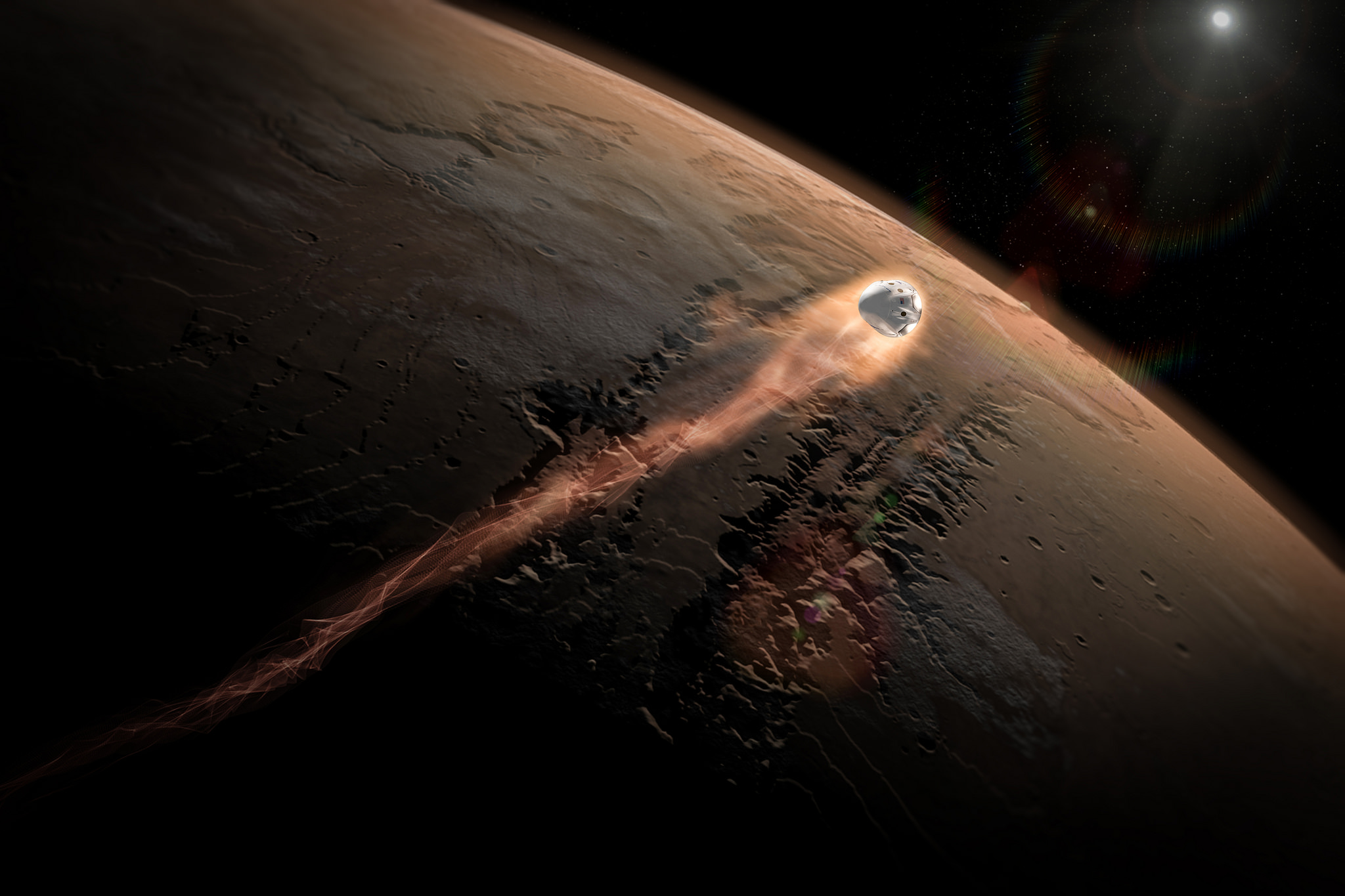
The concept of sending humans to Mars has been an exciting idea for decades, and the direction of space travel in the 21st century is finally presenting the possibility of actually making that happen. Of course, once everyone let the seriousness of such a journey sink in, the question of feasibility has inevitably come to the table for open discussion with the goal of finding realistic solutions.
It’s interesting enough to simply review the missions of all the Mars hopefuls (Part 1), but now that the reviews are in, it’s the details that are driving the discussion. After all, even the casual observer knows that deep space travel presents challenges such as long-term zero gravity and the ever-popular doom-and-gloom danger of cosmic radiation.
[Say that last one in a loud, booming voice for extra effect.]
Before breaking down any specifics, I want to acknowledge that there’s more than just a twelve-step program to getting to Mars (twelve being the obligatory “go-to” number). It requires an entire infrastructure of capabilities that build upon and support one another. However, I’m taking a leap of faith by assuming that inevitably anyone making a successful trip to Mars will have partnerships in place to tap into such an infrastructure. It’s the larger components of the specific missions that I’m focusing on here.
Outlining the Challenges for a Mission to Mars
NASA has a dedicated “Space Technology Mission Directorate” (STMD) charged with developing the capabilities needed to achieve the missions and goals NASA is given.
With the red planet as one of the big missions of the day (meaning Mars obviously, although Pluto has also been determined to be red), there’s no shortage of PowerPoints, panels, and interviews to source for what’s being worked on. I’ll follow their lead for discussion.
Transportation
First and foremost, in order to explore Mars, we’ve got to get there and (arguably) back. Depending on the length of stay and mission purpose, the cargo needs are going to play a part in the “how” part of this puzzle piece. Small stuff, no sweat (relative to general space traveler sweat levels). Big stuff? Now we’ve got issues.
Propulsion
Propulsion has been an interesting discussion to watch from the sidelines, mainly due to the debate over the types of systems available versus the types of systems thought to be needed. General mission discussions tend towards a six to eight month flight time each way plus a year and a half or so on the surface, but there are those advocating for shorter flight times to mitigate hazard exposure and reduce cargo needs.
Current rocket fuels can speed things along, but only at the expense of high fuel consumption. Nuclear fusion (and fission) systems are in the works which would theoretically reduce the flight time to Mars to approximately three months, but the timeframe needed to fully develop and test such new technologies isn’t a big crowd pleaser.
The methane-based nature of SpaceX’s Raptor engine for their speculated Mars Colonial Transport doesn’t really lend either way to this debate because using methane is a choice surrounding resource availability rather than power levels. Since methane can be harvested and manufactured on Mars, it reduces the need to carry as much fuel from Earth on missions, thus lowering costs. Methane-based fuel generation is also one of the key parts of the Mars Society’s “Mars Direct” proposal.
Entry, Descent, and Landing
Given the fact that we’ve sent several rovers to Mars already, it might be surprising that getting a craft from orbit to the Martian surface is actually a huge challenge. A quick survey of our recent history certainly makes the case for landing to be a non-issue, so what’s the deal?
Yes, we land heavy things on Earth all the time, but we do so with an atmosphere about 99% thicker than the one on Mars. The lack of air pressure and wind on Mars means that there isn’t any real air resistance to aid in slowing down a massive descending craft nor is there any wind to tap into for a glider or parachute to be very effective.
What about the moon?
There’s virtually no atmosphere there, either, yet we landed quite a bit of cargo during the Apollo program. That explanation would be gravity. The moon has less than half the gravity that Mars does, which is less than 20% that of Earth. The difference in power required to land a crew module on the moon vs. Mars could maybe be compared to landing a mini Falcon 9 with a micro drone onto a piece of plywood in the middle of a swimming pool versus dropping, say, a child-sized Tesla Model S. Maybe not, but it’s fun to think about. So cute…
In 2012, NASA landed the rover “Curiosity” on the Martian surface using a very complicated parachute-plus-propulsion crane system. The existence of such technology somewhat gives the impression that landing things on Mars is already a solved problem. If what we’re landing is about the mass of a small car, this impression is true, but if we are landing anything significantly larger, such as a capsule carrying humans for example, then the problem is still a problem as larger masses require greater counterforce to slow down their descent.
SpaceX Gives Back
SpaceX’s focus on developing propulsive landing systems is aiming to solve the problem of counterforce. This is actually an area where SpaceX is supporting NASA’s Journey to Mars (instead of the other way around) via the data obtained from their Falcon 9 landings to date. One of NASA’s proposed solutions is a “supersonic retropropulsion” system, meaning periodic firing of the engines on a craft to counter the speed resulting from a trip through the (small) Martian atmosphere. To date, NASA hasn’t been able to test this type of technology in an environment similar to what would be encountered on Mars whereas SpaceX has. By studying the results of SpaceX’s Falcon 9 first stage landings, NASA can use the information gathered for their retropropulsive system designs.
Back scratchers, unite!
Crew Systems
The crew ships under development for taking astronauts to Mars have a number of requirements to meet to be successful transports, and from the information available thus far, their progress seems to be moving along swimmingly. SpaceX’s Crew Dragon has been announced with photos and basic details provided, and NASA’s Orion capsule has enjoyed a marketing campaign providing numerous details for quite some time now.
The primary improvements in both capsule designs over the Apollo age seem to be more room, better heat shields, better software, and glass cockpits (i.e., touch screens). Crew Dragon can also hover (eventually landing) and blast off from its rocket transport in an emergency event. The aesthetics are pretty swank as well. Why isn’t there anything vastly different from what we’ve already done?
If it ain’t broke, don’t fix it.
Crew Cargo & Environmental Systems
Environmental systems and supplies to keep human travelers alive and (mostly) happy have been generally worked out via prior orbital missions, especially on the long-term International Space Station (ISS) ones. However, there are a few added “catches” that a mission to Mars throws in.
First, the ISS is able to maintain long-term human crews due to regular cargo resupply missions. The travel distance for Mars-bound astronauts will render such types of delivery schedules unavailable. No cargo deliveries mean carrying all the cargo required for the entire trip, something that generally demands multiple rocket launches for supply assembly before heading out.
Other than the higher expense of multiple launches, this seems to just be a matter of logistics and cost effectiveness rather than capability. SpaceX’s Falcon Heavy was certainly designed with these cargo requirements in mind considering the power packed into its engines.
Second, life support system technology has been developed and advanced over the years on the ISS, but it requires a lot of maintenance to upkeep. Perhaps the life support systems on the new crew capsules will endure for longer than the systems on the ISS as they have the data available to design around, but in the event that upkeep is just a fact of life that can’t be prevented, crews will surely undergo the training to perform repairs as needed as they are now.
As development in the space industry continues, these issues may become minimal. For instance, short-term resupply missions could eventually become available as travel time to Mars decreases with more efficient and powerful propulsion systems. The development of photon propulsion via lasers is ongoing, the goal being to accelerate around 220 pounds of unmanned spacecraft to 25% the speed of light for a three-day trip to Mars. That could almost translate into a sort of Mars-based Amazon Prime. I see what you’re up to, Jeff Bezos!
SpaceX also plans on making regular cargo missions to Mars a bi-annual affair, so as long as supplies and equipment can last for the 26-month(ish) window between launches, it’s Mars-certified.
Zero Gravity Impacts
When astronauts return from long-term zero gravity, their bodies have to acclimate after changes despite attempts to mitigate the effects through exercise regimens. If you’re just going from Earth to space and then Earth again, no big deal really. But going from Earth to space and then Mars? There won’t be a team of medical professionals ready to drag the astronauts out of the capsule and tell them to take it easy for a while.
That’s kind of an amusing image, actually. The Red Dragon capsule lands but everyone inside is all laid out looking like they are badly hungover from the prior night’s club hopping. Throw in some glitter for Instagram? Sorry, I’m digressing…
What exactly are the effects of long-term zero gravity on the human body? According to NASA, muscles (including the heart) can atrophy at a rate of 5% per week, bones at 1% per month, and about 22% of blood volume is lost. These are generally recoverable, but it takes about as long to recover a muscle as it did to lose it, and bone can take two to three years to grow back if it does at all. The lower Mars gravity would probably mean an easier recovery process, but there’s still a process involved and the entire crew is affected. Not even regular exercise can mimic all of the (needed) effects that gravity has on the body.
The concept of using a rotating space craft to mitigate this problem is seen so often in movies and space habitat designs that one might think it’s a “given” that some version of it will be used for Mars travel. In fact, The Mars Society’s “Mars Direct” plan even advocates for a rotating craft which uses the spent upper stage of the rocket as an anchor to spin the crew capsule around for artificial gravity simulation.
Since nothing looked like it would “spin” on the Dragon and Falcon Heavy media releases nor did there seem to be much room for a treadmill, I was really curious about what SpaceX’s answer to long term zero gravity was. From what I’ve read, it isn’t seen as a real problem or “show stopper”, if you will. Again, I’m missing a direct source to cite for any Elon or SpaceX comment on the issue, but from commentary around the web, it seems that the issue has surfaced in public discussions with no particular technology addressed to overcome it.
Perhaps this is one more thing we will see come September when SpaceX’s Mars Colonial Transporter plans are revealed. I can’t imagine that one hundred body-worn, space-traveling colonists wouldn’t be a problem needing to be addressed.
Surface Power
When it comes to any sort of space travel, solar seems to be one of the “go to” choices for power sourcing outside of propulsion. Unfortunately, when it comes to Mars exploration, solar power alone may not be enough. For one thing, Mars receives less than half the sunlight that Earth does, and most of that sunlight is only available in certain regions of the planet such as around the equator. Frequent light-blocking dust storms are also a problem. NASA’s STMD has outlined advanced batteries, regenerative fuel cells, fission nuclear systems, and solar arrays as the choice technologies for development in the area of surface power.
Now, I admit that I don’t have all the time in the world to watch every Elon Musk video in existence (although I do enjoy the convenience of a YouTube channel with nearly all of them compiled), but I haven’t had much luck finding original sources of either Elon or a SpaceX executive directly commenting on the subject of surface power. I’m sure something is out there either eluding me or that I’ve forgotten I’ve seen.
Crew Dragon uses solar arrays attached to its trunk during flight for power, but the trunk is jettisoned prior to reentry (or entry when talking about Mars). I could make an educated guess based on the connections between Elon Musk and Solar City, Tesla, and the methane-based Raptor engines to presuppose that solar power, advanced batteries, and methane fuel generation are part of SpaceX’s surface power plans, but in the end it’s just a guess. Also, if Raptor is using a methane-based fuel because it can be resourced outside of Earth, I’d imagine that surface power would tie into that same manufacturing capability.
Mars One plans to utilize solar power for its surface power needs, specifically “thin film solar photovoltaic panels”. There isn’t much detail about their required panel size available, only that they should have the ability to be rolled up and transported elsewhere if need be. Finally, as I mentioned previously, the “Mars Direct” plan advocates tapping into fuel generation structures that manufactures a Methane-Oxygen bi-propellant.
Overall, it seems everyone is likely on a similar page regarding power sources – nothing crazy or unheard of, unless you think nuclear anything is too risky.
Coming Up on Countdown to Mars…
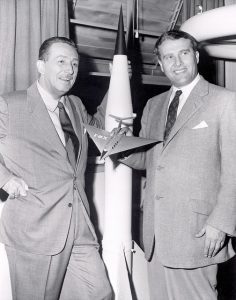
Wernher von Braun and Walt Disney | Credit: NASA on The Commons
Cosmic space radiation! There’s so much on this topic, it’s worth an entire piece on its own. Spoiler alert: Elon doesn’t seem to be worried about that issue. Why not?
Also, stay tuned for a (theoretical) discussion on future Martian government…
Did you know that Werner von Braun had a fictional tale of a Martian society wherein the elected Martian leader was called “The Elon”? It’s almost as though he really did take a trip on that Nazi time traveling bell thing…
News
SpaceX’s Crew-11 mission targets July 31 launch amid tight ISS schedule
The flight will lift off from Launch Complex 39A at Kennedy Space Center in Florida.
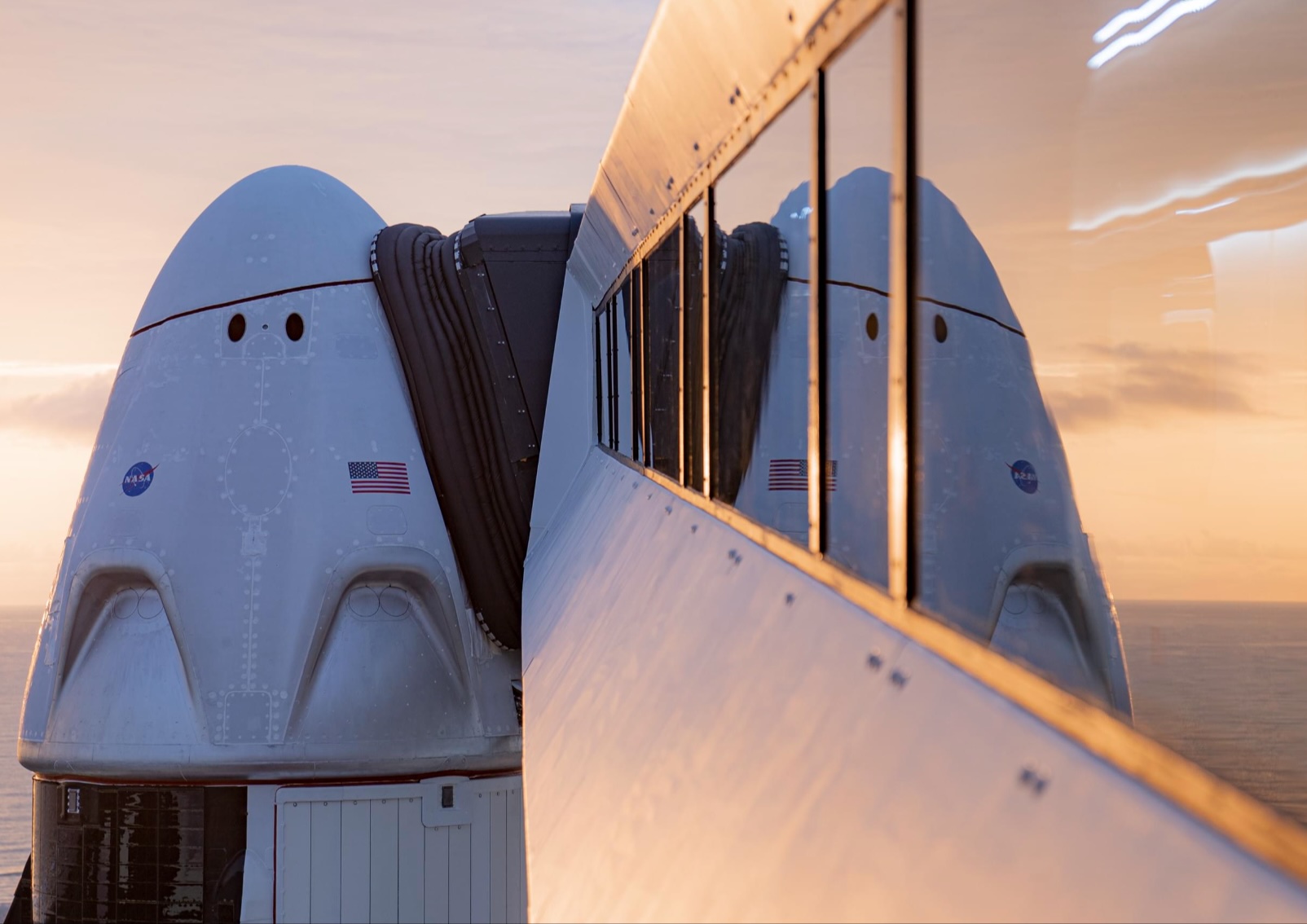
NASA and SpaceX are targeting July 31 for the launch of Crew-11, the next crewed mission to the International Space Station (ISS). The flight will lift off from Launch Complex 39A at Kennedy Space Center in Florida, using the Crew Dragon Endeavour and a Falcon 9 booster.
Crew Dragon Endeavour returns
Crew-11 will be the sixth flight for Endeavour, making it SpaceX’s most experienced crew vehicle to date. According to SpaceX’s director of Dragon mission management, Sarah Walker, Endeavour has already carried 18 astronauts representing eight countries since its first mission with NASA’s Bob Behnken and Doug Hurley in 2020, as noted in an MSN report.
“This Dragon spacecraft has successfully flown 18 crew members representing eight countries to space already, starting with (NASA astronauts) Bob (Behnken) and Doug (Hurley) in 2020, when it returned human spaceflight capabilities to the United States for the first time since the shuttle retired in July of 2011,” Walker said.
For this mission, Endeavour will debut SpaceX’s upgraded drogue 3.1 parachutes, designed to further enhance reentry safety. The parachutes are part of SpaceX’s ongoing improvements to its human-rated spacecraft, and Crew-11 will serve as their first operational test.
The Falcon 9 booster supporting this launch is core B1094, which has launched in two previous Starlink missions, as well as the private Ax-4 mission on June 25, as noted in a Space.com report.
The four-members of Crew-11 are NASA astronauts Zena Cardman and Mike Fincke, as well as Japan’s Kimiya Yui and Russia’s Oleg Platonov.
Tight launch timing
Crew-11 is slated to arrive at the ISS just as NASA coordinates a sequence of missions, including the departure of Crew-10 and the arrival of SpaceX’s CRS-33 mission. NASA’s Bill Spetch emphasized the need for careful planning amid limited launch resources, noting the importance of maintaining station altitude and resupply cadence.
“Providing multiple methods for us to maintain the station altitude is critically important as we continue to operate and get the most use out of our limited launch resources that we do have. We’re really looking forward to demonstrating that capability with (CRS-33) showing up after we get through the Crew-11 and Crew-10 handover,” Spetch stated.
News
SpaceX launches Ax-4 mission to the ISS with international crew
The SpaceX Falcon 9 launched Axiom’s Ax-4 mission to ISS. Ax-4 crew will conduct 60+ science experiments during a 14-day stay on the ISS.
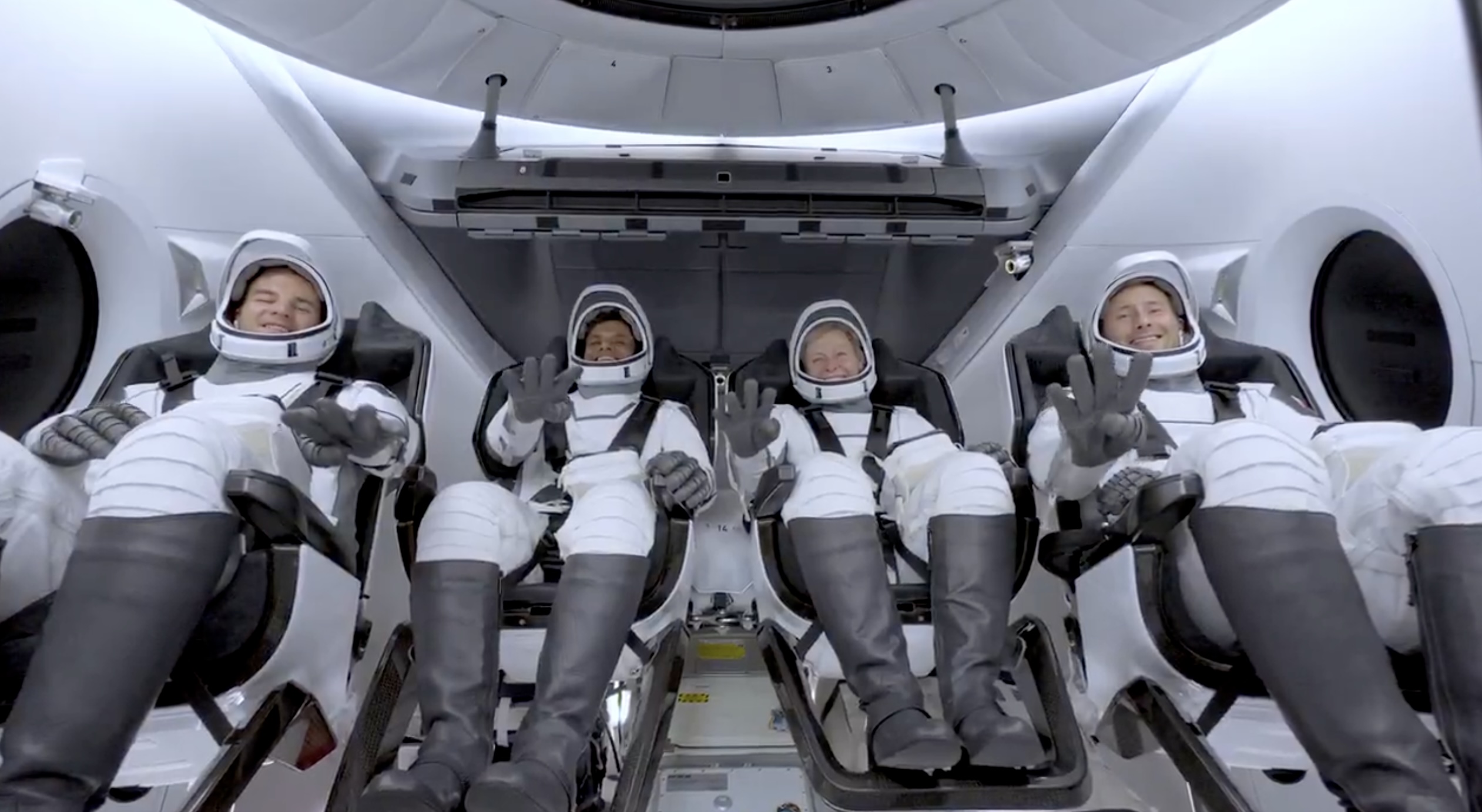
SpaceX launched the Falcon 9 rocket kickstarting Axiom Space’s Ax-4 mission to the International Space Station (ISS). Axiom’s Ax-4 mission is led by a historic international crew and lifted off from Kennedy Space Center’s Launch Complex 39A at 2:31 a.m. ET on June 25, 2025.
The Ax-4 crew is set to dock with the ISS around 7 a.m. ET on Thursday, June 26, 2025. Axiom Space, a Houston-based commercial space company, coordinated the mission with SpaceX for transportation and NASA for ISS access, with support from the European Space Agency and the astronauts’ governments.
The Ax-4 mission marks a milestone in global space collaboration. The Ax-4 crew, commanded by U.S. astronaut Peggy Whitson, includes Shubhanshu Shukla from India as the pilot, alongside mission specialists Sławosz Uznański-Wiśniewski from Poland and Tibor Kapu from Hungary.
“The trip marks the return to human spaceflight for those countries — their first government-sponsored flights in more than 40 years,” Axiom noted.
Shukla’s participation aligns with India’s Gaganyaan program planned for 2027. He is the first Indian astronaut to visit the ISS since Rakesh Sharma in 1984.
Axiom’s Ax-4 mission marks SpaceX’s 18th human spaceflight. The mission employs a Crew Dragon capsule atop a Falcon 9 rocket, designed with a launch escape system and “two-fault tolerant” for enhanced safety. The Axiom mission faced a few delays due to weather, a Falcon 9 leak, and an ISS Zvezda module leak investigation by NASA and Roscosmos before the recent successful launch.
As the crew prepares to execute its scientific objectives, SpaceX’s Ax-4 mission paves the way for a new era of inclusive space research, inspiring future generations and solidifying collaborative ties in the cosmos. During the Ax-4 crew’s 14-day stay in the ISS, the astronauts will conduct nearly 60 experiments.
“We’ll be conducting research that spans biology, material, and physical sciences as well as technology demonstrations,” said Whitson. “We’ll also be engaging with students around the world, sharing our experience and inspiring the next generation of explorers.”
SpaceX’s Ax-4 mission highlights Axiom’s role in advancing commercial spaceflight and fostering international partnerships. The mission strengthens global space exploration efforts by enabling historic spaceflight returns for India, Poland, and Hungary.
News
Starlink Cellular’s T-Mobile service to grow with third-party app data
From Oct 2025, T-Satellite will enable third-party apps in dead zones! WhatsApp, X, AccuWeather + more coming soon.
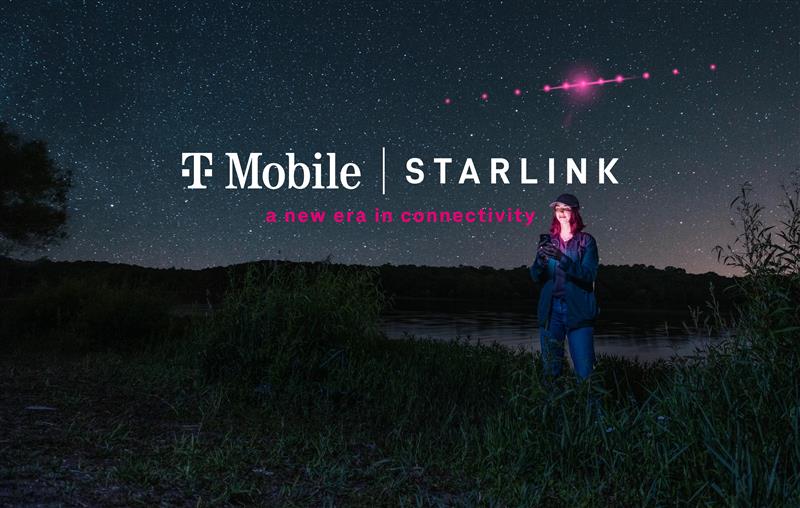
Starlink Cellular’s T-Mobile service will expand with third-party app data support starting in October, enhancing connectivity in cellular dead zones.
T-Mobile’s T-Satellite, supported by Starlink, launches officially on July 23. Following its launch, T-Mobile’s Starlink Cellular service will enable data access for third-party apps like WhatsApp, X, Google, Apple, AccuWeather, and AllTrails on October 1, 2025.
T-Mobile’s Starlink Cellular is currently in free beta. T-Satellite will add MMS support for Android phones on July 23, with iPhone support to follow. MMS support allows users to send images and audio clips alongside texts. By October, T-Mobile will extend emergency texting to all mobile users with compatible phones, beyond just T-Mobile customers, building on its existing 911 texting capability. The carrier also provides developer tools to help app makers integrate their software with T-Satellite’s data service, with plans to grow the supported app list.
T-Mobile announced these updates during an event celebrating an Ookla award naming it the best U.S. phone network, a remarkable turnaround from its last-place ranking a decade ago.
“We not only dream about going from worst to best, we actually do it. We’re a good two years ahead of Verizon and AT&T, and I believe that lead is going to grow,” said T-Mobile’s Chief Operating Officer Srini Gopalan.
T-Mobile unveiled two promotions for its Starlink Cellular services to attract new subscribers. A free DoorDash DashPass membership, valued at $10/month, will be included with popular plans like Experience Beyond and Experience More, offering reduced delivery and service fees. Meanwhile, the Easy Upgrade promotion targets Verizon customers by paying off their phone balances and providing flagship devices like the iPhone 16, Galaxy S25, or Pixel 9.
T-Mobile’s collaboration with SpaceX’s Starlink Cellular leverages orbiting satellites to deliver connectivity where traditional networks fail, particularly in remote areas. Supporting third-party apps underscores T-Mobile’s commitment to enhancing user experiences through innovative partnerships. As T-Satellite’s capabilities grow, including broader app integration and emergency access, T-Mobile is poised to strengthen its lead in the U.S. wireless market.
By combining Starlink’s satellite technology with strategic promotions, T-Mobile is redefining mobile connectivity. The upcoming third-party app data support and official T-Satellite launch mark a significant step toward seamless communication, positioning T-Mobile as a trailblazer in next-generation wireless services.
-
Elon Musk2 weeks ago
Tesla investors will be shocked by Jim Cramer’s latest assessment
-
News2 days ago
Tesla debuts hands-free Grok AI with update 2025.26: What you need to know
-
Elon Musk4 days ago
xAI launches Grok 4 with new $300/month SuperGrok Heavy subscription
-
Elon Musk6 days ago
Elon Musk confirms Grok 4 launch on July 9 with livestream event
-
News1 week ago
Tesla Model 3 ranks as the safest new car in Europe for 2025, per Euro NCAP tests
-
Elon Musk2 weeks ago
xAI’s Memphis data center receives air permit despite community criticism
-
News4 days ago
Tesla begins Robotaxi certification push in Arizona: report
-
Elon Musk2 weeks ago
Tesla scrambles after Musk sidekick exit, CEO takes over sales